Glucosamine (GlcN) is an aminomonosaccharide that is produced naturally in the body from glucose and an amine. As a precursor to proteoglycans and glycosaminoglycans, the building blocks of cartilage, GlcN plays a key role in the maintenance of joint health. Thus, GlcN is often taken in supplement form as glucosamine sulfate for the management of osteoarthritis; it has also been used in pet food and cosmetics applications. Presently, the commercial production of GlcN involves the hydrolysis of chitin or chitosan from shellfish exoskeletons, but this process requires high temperature and substantial amounts of concentrated acid, which is not environmentally friendly. In addition, as the current production method utilizes shellfish byproducts, the resulting supplement may not be appropriate for individuals with shellfish allergies nor those following a vegan diet. As a result, enzymatic synthesis of GlcN from N-acetylglucosamine (GlcNac) using diacetylchitobiose deacetylase (Dac) is becoming a more attractive option. The goal of this study, conducted by Sun et al at Jiangnan University in Wuxi, China, was to use directed evolution to improve the activity of Dac for the industrial production of GlcN.
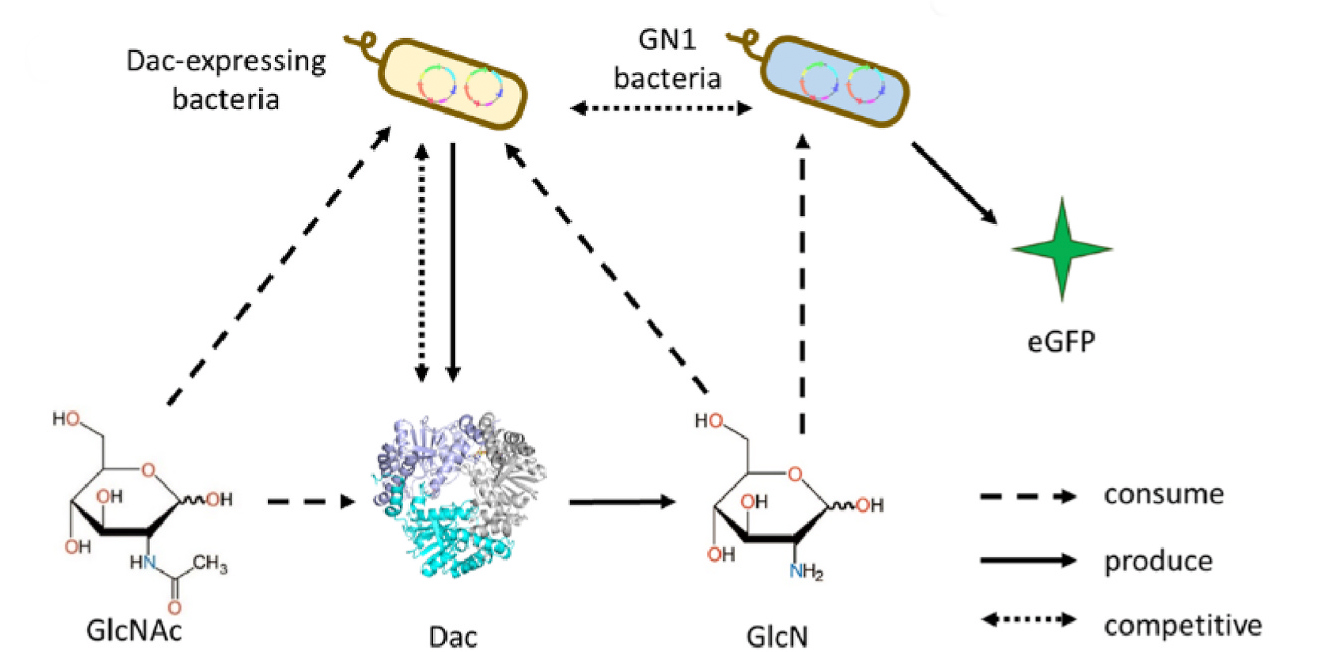
To conduct an effective directed evolution campaign, it is necessary to screen a large number of variants for enhanced catalytic activity. In this case, no functional assay had yet been established for high-throughput droplet sorting based on Dac activity. To that end, the authors engineered a novel bacteria-based biosensor that would enable the detection of superior Dac mutants through fluorescence-activated droplet sorting (FADS). They constructed a GlcN-detecting strain (GN1) of Bacillus subtilis that lacked the main catabolic pathway for creating glucosamine-6-phosphate (GlcN6P) and so will take up GlcN from the environment to produce GlcN6P. They inserted a genetic cassette containing eGFP under the control of the GlcN6P-responsive promoter, so that the production of GlcN6P from GlcN would result in a fluorescent signal. The GlcNAc transporter was also deleted from GN1; thus, the fluorescent signal could be directly related to GlcN uptake from the environment. Experiments designed to characterize the ability of strain GN1 as a GlcN biosensor showed that eGFP expression was positively correlated to GlcN concentration in the range of 0-1.5 g/L, and that GlcNAc did not influence these results. Since GlcN concentration is a product of Dac catalysis, GN1 would produce a stronger fluorescence signal in the presence of a Dac variant with higher catalytic activity, thus enabling the in vitro selection of improved Dac variants by FADS.
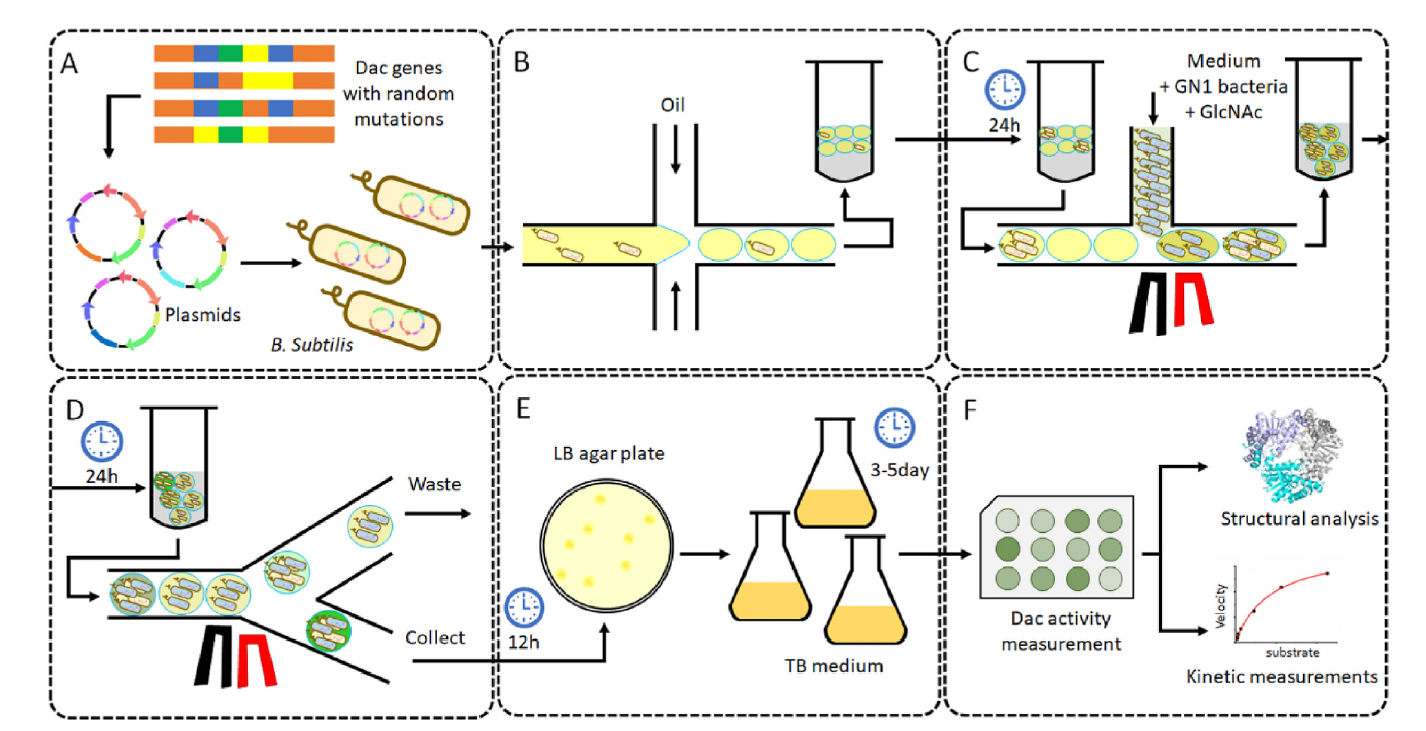
To begin the directed evolution campaign, a Dac mutant library was generated by using error-prone polymerase chain reaction, with Dac mutant R157T serving as a template. Dac mutant R157T had been generated in a previous work by the authors and already exhibits higher activity than wild type Dac. Single bacteria containing the new Dac variants were encapsulated in droplets and incubated for 24 h. Each droplet was then injected with GlcN detecting solution, which contained the GN1 biosensor strain and GlcNAc, and incubated again for 24 h. The incubated droplets were then injected into the microfluidics device for high-throughput functional screening using FADS. The selection criteria were configured to collect the top 1% Dac-expressing bacteria based on Dac activity. Sorted bacteria were released from the droplets and plated on LB agar for 12 h, after which time individual colonies were isolated for 3-6 days of fermentation. Ten Dac variants were identified that exhibited higher catalytic activity than R157T.
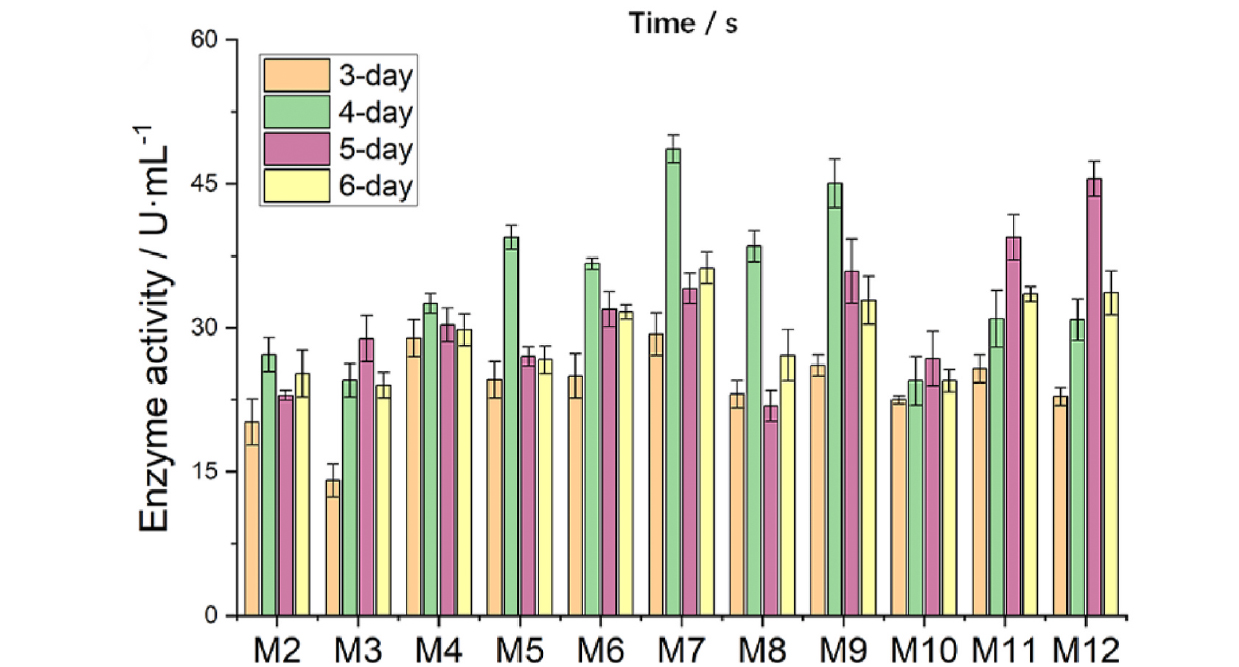
Strain M7 was shown to contain the highest performing Dac variant among all positive mutants, with a catalytic activity 1.8 times that of R157T. The kinetic constant of M7 (755.9 ± 24.0 µM/s) was double that of R157T (368.8 ± 19.2 µM/s), confirming the greater catalytic efficiency of the selected variant. The authors determined that variant M7 contained two additional mutant sites in comparison to R157T. The first mutant site, S60I, was far from the catalytic pocket of GlcNAc, whereas the second mutant site, F168S, was close to the catalytic pocket, suggesting the latter as the primary contributor to the enhanced catalytic activity of Dac. As more progress is needed in optimizing the enzymatic production of GlcN, the variants identified here, along with the creation of the bacteria-based GlcN biosensor, will be useful for future work towards this purpose. In addition, the biosensor could be converted to measure other metabolic products and facilitate the directed evolution of other industrially relevant enzymes via high-throughput fluorescence-activated droplet sorting.