Fasciano S & Wang S, University of New Haven . Summary prepared by Andréa Covey for Atrandi Biosciences.
Artificial cells are synthetic, cell-like structures that are engineered to replicate essential biological functions. The goal is to create a simplified version of a living cell that can support the study of specific cellular functions free from unrelated processes. In this context, research involving artificial cells provides a valuable pathway to delve into the complexities of membrane protein interactions, cell signaling, and gene expression. Artificial cell studies also hold significant potential for biomaterial synthesis and drug development. Droplet microfluidics technology is becoming the preferred methodology for the bottom-up synthesis of artificial cells, due to its remarkable precision in creating uniform compartments and its high-throughput capabilities. In a recent review for SLAS Technology, Fasciano and Wang from the University of New Haven describe how droplet-based microfluidic technologies are being used to create artificial cells. They also discuss the application of artificial cells to the study of fundamental biological processes, such as transcription, translation, and membrane interactions.
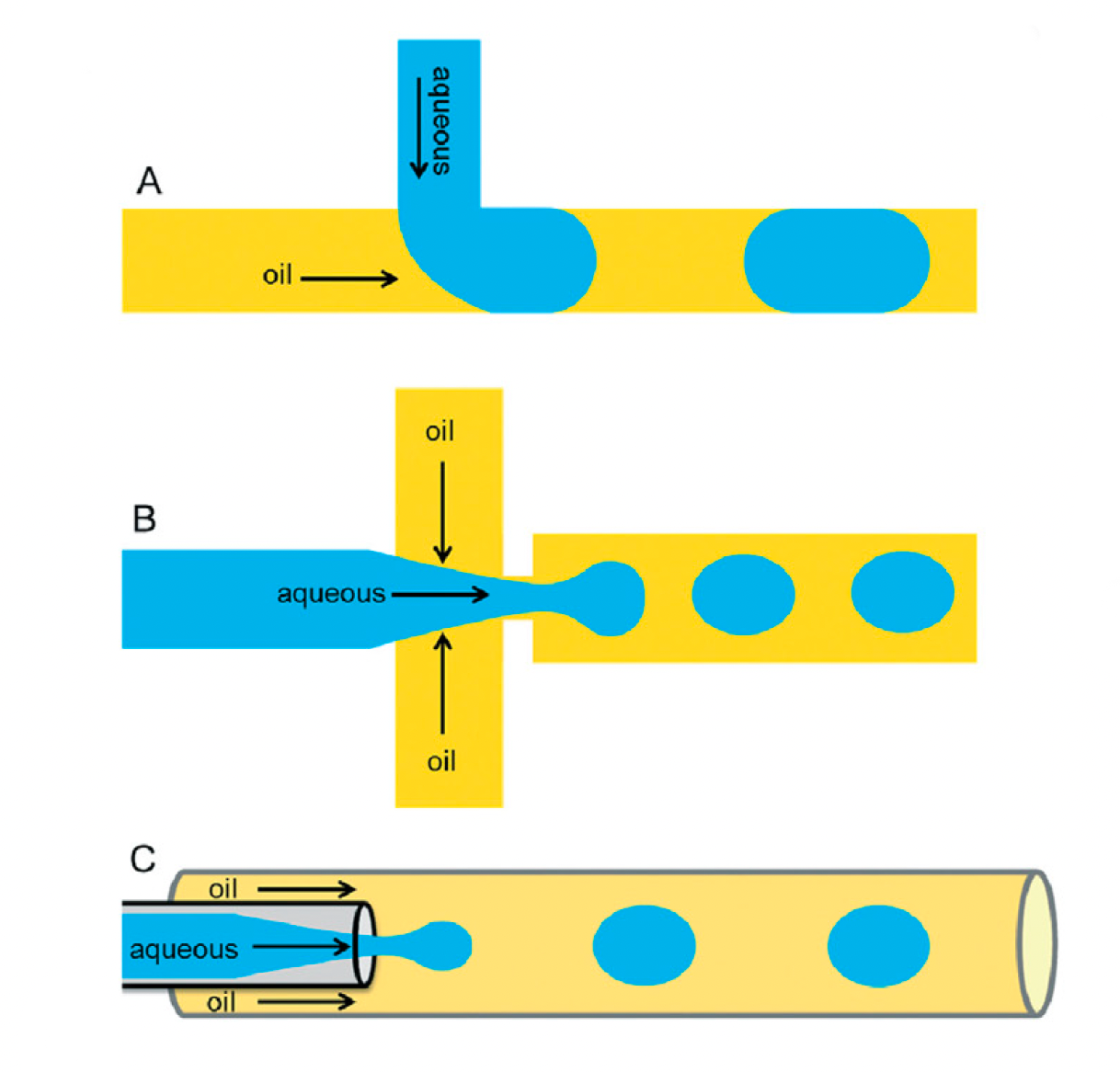
Droplet microfluidic devices are capable of manipulating picoliter- to nanoliter-sized volumes of liquid into uniform compartments with a spherical shape. The resulting droplets offer a stable and easily controllable microenvironment for engineering artificial cell components. There are several types of microfluidic devices that can be used for droplet generation, including devices with T-junction, flow-focusing, or co-flowing geometries. In all cases, the fundamental principle involves harnessing the behavior of immiscible phases within microscale channels, and all three types of devices can be used to generate many droplets in a short period of time. For the fabrication of artificial cells, however, both flow-focusing and co-flowing microfluidic devices offer advantages over T-junction microfluidic devices because they can encapsulate multiple compartments within a single droplet. This is an essential capability when replicating natural cells with multiple organelles.

For an artificial cell to faithfully replicate cellular properties and behaviors (e.g., cell-cell communication, the uptake of nutrients, the removal of wastes), it must be surrounded by a lipid bilayer membrane with an asymmetrical composition and arrangement of lipids, proteins, and other molecules on the inner and outer layers. One way to produce a lipid bilayer membrane using droplet microfluidics is via water/oil/water double emulsions. As lipids have been dissolved in the intermediate oil phase, once the oil is removed from the droplets, lipid bilayers remain, effectively forming artificial cell-like membranes. Another technique involves generating two separate water-in-oil droplets and bringing them into contact. The lipids at the interface interact and rearrange, forming one droplet with a bilayer membrane. Finally, droplet microfluidics can be used to produce giant unilamellar vesicles (GUVs), which closely resemble the size and shape of natural eukaryotic cells. Stabilized GUVs can even act as subcompartments where biomolecules can be introduced for further studies.
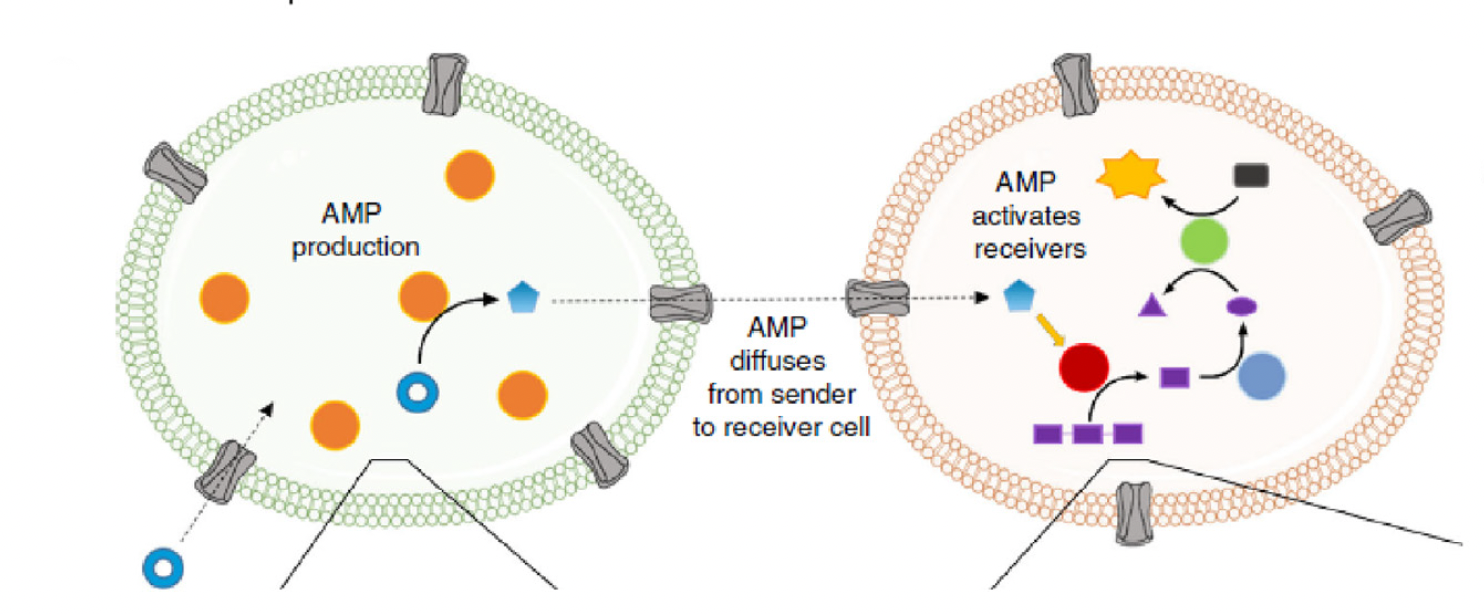
Artificial cells are an excellent platform for exploring fundamental biological processes, such as transcription, translation, and membrane interactions. For example, artificial cells were used by Zhang et al (2020) to examine light-sensitive molecular switches and by Jia et al (2019) to explore how temperature-sensitive non-coding RNA sequences enable protein synthesis at specific temperatures. Several groups have engineered artificial cells capable of intercellular communication (e.g., Adamala et al 2017, Buddingh’ et al 2020, Yang et al 2020). Droplet microfluidics technology, with its high-throughput capabilities, can help to facilitate large-scale experiments by rapidly generating thousands of artificial cells with consistent sizes and contents. Future developments will likely involve creating artificial cells with enhanced biomimicry and functional integration that could eventually provide insights into human health, disease, and the development of novel therapeutics.
- Fasciano S, Wang S. Recent advances of droplet-based microfluidics for engineering artificial cells. SLAS Technol. 2023 May 26:S2472-6303(23)00034-1. doi: 10.1016/j.slast.2023.05.002. Epub ahead of print. PMID: 37245659.
- Zhang P, Yang J, Cho E, Lu Y. Bringing Light into Cell-Free Expression. ACS Synth Biol. 2020 Aug 21;9(8):2144-2153. doi: 10.1021/acssynbio.0c00211. Epub 2020 Jul 15. PMID: 32603590.
- Jia H, Heymann M, Härtel T, Kai L, Schwille P. Temperature-sensitive protein expression in protocells. Chem Commun (Camb). 2019 May 30;55(45):6421-6424. doi: 10.1039/c9cc02734c. PMID: 31094370.
- Adamala KP, Martin-Alarcon DA, Guthrie-Honea KR, Boyden ES. Engineering genetic circuit interactions within and between synthetic minimal cells. Nat Chem. 2017 May;9(5):431-439. doi: 10.1038/nchem.2644. Epub 2016 Nov 14. PMID: 28430194; PMCID: PMC5407321.
- Buddingh' BC, Elzinga J, van Hest JCM. Intercellular communication between artificial cells by allosteric amplification of a molecular signal. Nat Commun. 2020 Apr 3;11(1):1652. doi: 10.1038/s41467-020-15482-8. PMID: 32246068; PMCID: PMC7125153.
- Yang Q, Guo Z, Liu H, Peng R, Xu L, Bi C, He Y, Liu Q, Tan W. A Cascade Signaling Network between Artificial Cells Switching Activity of Synthetic Transmembrane Channels. J Am Chem Soc. 2021 Jan 13;143(1):232-240. doi: 10.1021/jacs.0c09558. Epub 2020 Dec 28. PMID: 33356224.