The development of 3D cancer models has significantly reduced the time, cost, and labor involved in the drug discovery pipeline by accelerating the design, discovery, and validation of potential targets and drug compounds. The use of engineered matrices and biomimetic materials has become more relevant, providing close recapitulation of the tumor microenvironment. Although spheroidal cell aggregate models have been established as more accurate than 2D models, they have limitations regarding production consistency, control, and flexibility. Many of these platforms are also not scalable for use in multiwell-plate drug-testing assays. However, microfluidic fabrication technologies can be used to integrate the advantages of spheroid drug screening platforms with tissue engineering to control and manipulate the cellular microenvironment. While most cancer microencapsulation studies have demonstrated validation of the hydrogels, additional steps are needed to move toward the use of cancer hydrogels in high-throughput screening. Furthermore, the utilization of droplet microfluidics for constructing engineered cancer microtissues with biomaterials that better resemble the tumor microenvironment will improve drug screening for metastatic and invasive cancer cell lines. Here, Seeto et al. generated hybrid hydrogels with PEG (polyethylene glycol) conjugated to fibrinogen (PF hydrogels) to study metastatic breast cancer drug screening in an automated fashion.
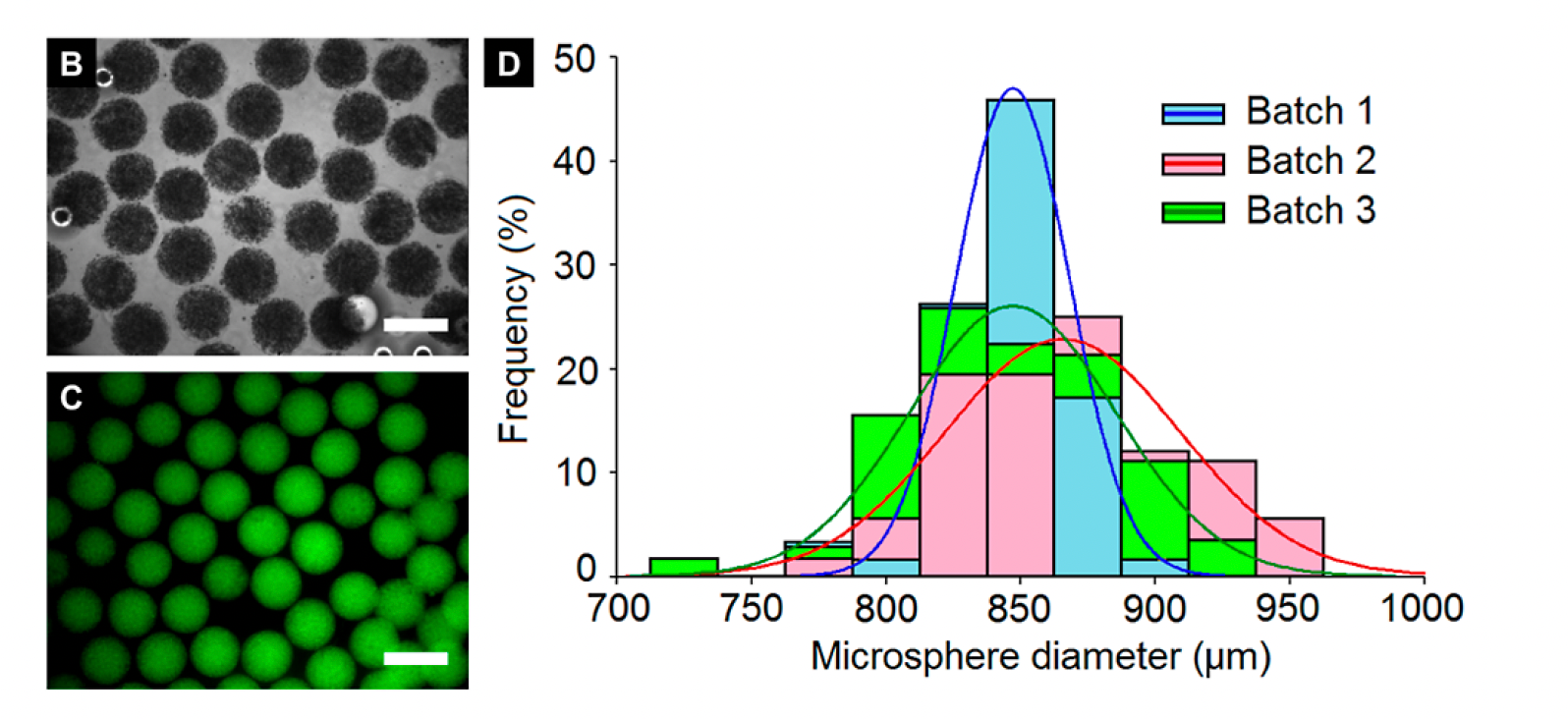
A modified T-junction was used to pump PF hydrogel and mineral oil in separate syringes to form hydrogel microspheres. In this study, the authors conjugated poly(ethylene glycol diacrylate) (PEGDA) and fibrinogen (PEG-fibrinogen, PF) to generate uniform microspheres encapsulating breast cancer cells of two cell lines (MCF7 and MDA-MB-231) to culture 3D tumors. Microsphere quantification immediately following post-fabrication demonstrated that microspheres were uniformed within a batch and across batches where most microspheres were ~850-900 µm. Not only did droplet microfluidics were able to generate highly uniformed encapsulated spheroids, but this finding also highlights the potential for hydrogels to be in the sub-millimeter range. This is an important finding because previous studies in cancer microtissue engineering with alginate hydrogels are approximately 250 µm in size, which limits the number of cancer cells that can be incorporated and range of responses that can be detected in downstream drug screening.
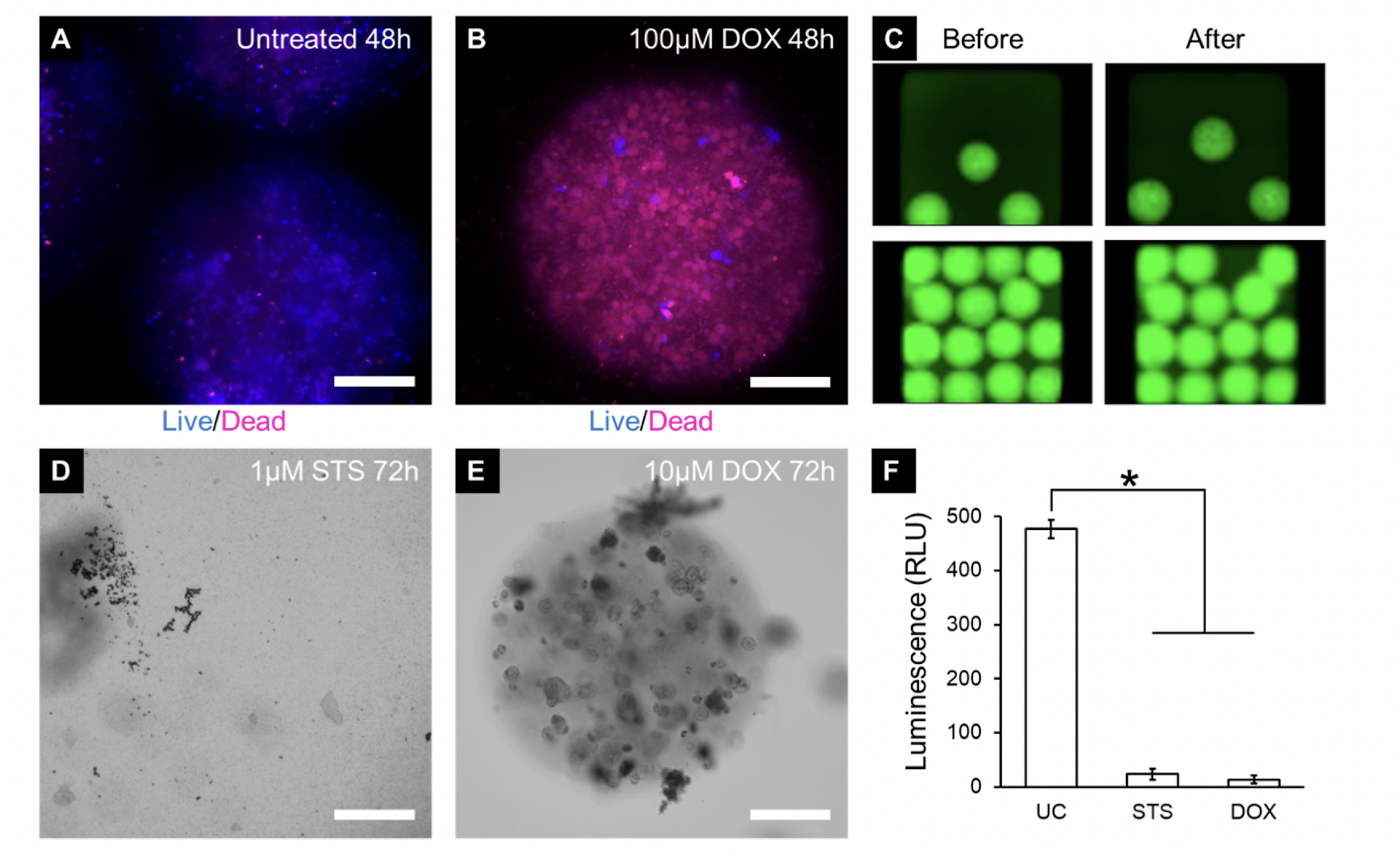
The authors next assessed the feasibility of using microspheres for automated drug testing on cancer cells in 384-well plates. The microspheres, containing MDA-MB-231 metastatic breast cancer cells, were successfully shipped overnight while maintaining cell viability. This suggests that cancer microspheres encapsulated in PF hydrogels were extremely stable. A 384-well pin tool device (with pins in 457 µm in diameter) was then used to administer drugs to microspheres without disruption of the microsphere shape and viability. Two anticancer agents were tested: 1) doxorubicin, which slows and stops the growth of cancer cells by targeting topoisomerase 2, and 2) staurosporine, which inhibits cancer cell growth by activating the intrinsic apoptotic pathways. Over a period of 72 hours, the microspheres treated with 1 µM staurosporine underwent complete degradation. On the other hand, the microspheres treated with 10µM doxorubicin remained mostly intact, but the cells contained within them displayed signs of cellular damage. The degradation observed in the staurosporine-treated microspheres may be attributed to the complete degradation of cancer cells, which in turn releases various nonspecific proteinases capable of breaking down the fibrinogen component of the PF hydrogels. In contrast, the doxorubicin-treated microspheres remained intact as the cells were not completely degraded, preventing the release of proteinases. Quantitative analysis revealed that both drug treatments significantly decreased cell viability compared to the untreated control group. These results findings suggest that the microspheres generated in this manner enabled high-throughput cancer drug screening.
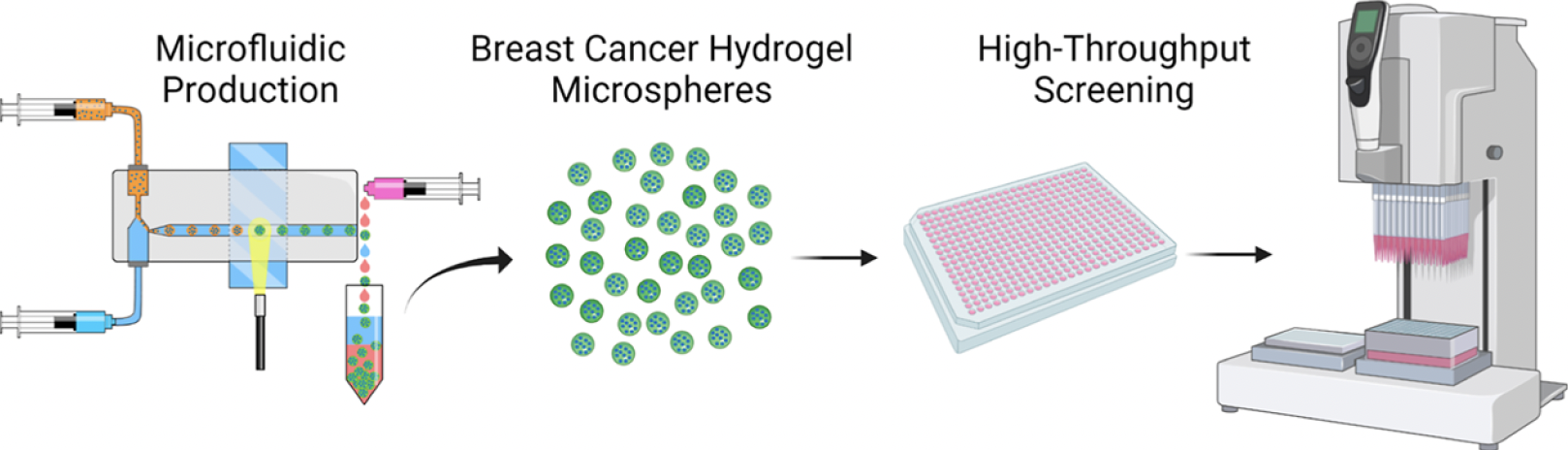
Although many studies have successfully validated the use of hydrogels for microencapsulation of cancer cells, further progress is required to enable the utilization of cancer hydrogels in high-throughput screening. These steps may include optimizing the encapsulation efficiency, enhancing the long-term stability of the hydrogels, and validating their ability to accurately recapitulate the tumor microenvironment and drug response in a high-throughput setting. In this study, the authors employed a droplet microfluidic system to generate uniform cancer microspheres efficiently and consistently with high cell densities. This microfluidic system allowed them to utilize tissue engineering techniques to construct engineered cancer tissues in a spheroidal shape, which could be readily integrated into existing assays that utilize spheroidal cell aggregates. The hydrogel microspheres they fabricated supported the 3D culture of breast cancer cells for at least 14 days, exhibiting cell-type-specific variations in morphology, proliferation, metabolic activity, ultrastructure, microsphere size distribution, and overall stiffness. Most importantly, the cancer microspheres were also compatible with an automated liquid handling system for drug administration and displayed appropriate drug responses post-treatment. By offering a more physiologically relevant drug response in a cost-effective and high-throughput manner, the cancer microspheres encapsulated in PF hydrogels have the potential to enhance the translational success of drug candidates by providing more accurate in vitro predictions of in vivo drug efficacy. Furthermore, this cancer microsphere platform opens doors for further investigations into cell-cell and cell-matrix interactions, tumorigenic processes, and disease progression.